Task Force 4: Refuelling Growth—Clean Energy and Green Transitions
Abstract
Electricity is crucial in ensuring optimum healthcare, education, quality of life, and sustained economic development. As countries embed clean energy and move to a standard all-round electrification practice, the health of the power system assumes greater significance. However, power system reliability is threatened by two factors: the variability of generation sources to facilitate the clean energy transition; and climate-induced extreme weather events that can lead to cascading impacts through the electricity value chain.
Not accounting for climate risks can burden or delay the pursuit of the clean energy transition. Further, there is a need for diversified supply chains to hedge against material availability risk, and ensure circular economic frameworks to maximise resource value.
The G20 should consider the following actions to build power sector resilience within the ambit of its wider international cooperation and knowledge-sharing work:
- Include resilience in long-term power-sector planning
- Invest in grid-hardening measures
- Leverage distributed clean energy technologies and grid digitalisation
- Invest in energy storage ecosystems that provide balancing services and flexibility
1. The Challenge
Climate change is a ‘threat multiplier’ due to its compounding effect on risks and a system’s exposure to high-impact, low-probability events. The power sector is vulnerable to climate change, thus adding a new dimension to the risks the sector faces. Power sector resilience is the ability of modern power systems to withstand extraordinary events, rapidly recover from such events, and learn to adapt to their operations such that the functionality of the whole system improves.
Increasing climate-induced extreme-weather events and the expanding extent of the electricity sector—both in terms of growing customers and the sectors to be electrified—stand to concentrate the risk of a system-wide disruption on electricity as a resource and the grid that transmits it (see Figure 1). Renewable energy (RE) assets and infrastructure, while contributing to climate change mitigation, are not immune to physical damage from climate-induced extreme-weather events either.
Thus, without planning for a ‘resilient’ power sector, sustainable development remains at risk. By negatively impacting economic productivity, increasing power outages are linked to a reduction in the per capita income of households.[1] At the same time, inadequacy of data and uncertainty associated with climate impacts enhance the complexity of planning within the power sector.
The current power sector infrastructure is inadequately equipped to onboard RE and manage the associated variability without flexibility of resources. These conditions are together leading to an overhaul of the incumbent system of transmitting power from a central point of generation to distributed end consumers.
Figure 1: Cascading impact on the electricity value chain due to climate-induced extreme-weather events

*Not exhaustive
Source: Powering Through: A Climate Resilient Future (RMI and RMI India, 2021)[2]
2. The G20’s Role
Ensuring electricity security and reliability is a growing concern for G20 member states, especially as per capita electricity demand in the group has risen 53.38 percent since 2000.[3] Failure to plan for resilience can impose costs of repair and reconstruction on local administrations, affect critical services and, by extension, communities. In this context, the G20 should recognise the role of building power sector resilience in advancing energy security, net zero goals, and societal benefits.
Power sector resilience assumes an international essence as multilateral collaborations pursuing round-the-clock clean-energy sharing via transnational supergrids, such as the One Sun One World One Grid initiative, are beginning to take shape.[4] Just like extreme weather events do not adhere to national boundaries, the implied risk will notably impact regionally integrated grids.
As an international consortium, the G20 is committed to improving energy security to support energy transitions through collaboration, as captured in the G20 Naples Principles.[5] Alignment within the group on employing power sector resilience as a crucial energy transition strategy will help build a collective understanding and more visibility around incorporating novel technologies and business models in power systems. With some of the world’s strongest economies as its members, the G20 can effectively model and mainstream action on power sector resilience regionally and globally.
3. Recommendations to the G20
Include resilience in long-term power-sector planning
Actions
- Share granular climate risk data and expertise so they may be processed for the benefit of power sector planners, including utilities and policymakers
- Explore non-wires alternative (NWA) projects that can replace traditional physical infrastructure needs
- Convene in bilateral or multilateral groupings within the G20 to conduct joint planning exercises, training and capacity building, and test pilots as required
Rationale
Long-term power planning entails outlining the right power mix, infrastructure, and associated costs and benefits while accounting for changing future electricity requirements and other stressors emanating from economic, geopolitical or weather-related risks.[6] Climate change, which operates on longer time scales and larger geographical scales than ‘weather’, is a new source of risks that the power sector faces.[7]
Climate impact manifests in different types of extreme weather events and slow changes over time. Not only are 100-year events becoming more frequent and unpredictable but the current infrastructure does not account for risks that previously occurred only once in a century. For example, in 2022, South Asia recorded higher than standard summer temperatures when heatwaves swept across north and central India and its neighbouring countries. According to climate scientists, the frequency of these heatwaves has increased from once every 50 years in a world without climate change impact to roughly once every four years in the current climate scenario.[8] The unexpected rise in peak demand to beat the heat has left power utilities underprepared, resulting in power outages that affected needs as diverse as cooling and irrigation.[9]
Quality data on climate change impact and access to appropriate tools to interpret such data can go a long way in helping power sector planners and utilities. The right datasets can help ascertain interventions to meet peak demand such as demand flexibility, energy efficiency and RE capacity that itself is affected by the forces of climate change. Further, suitable data can also help in better asset management and identification of locations where power infrastructure warrants retrofitting and where new, more resilient infrastructure can be built.[10] However, such decision-making is difficult as the data around climate risks comes with uncertainties. Long-term climate forecasts have lower reliability than short-term weather forecasts. Developing capabilities such as advanced RE and electricity-demand forecasting can help address such variability and uncertainty. For improved forecasts, it is essential to build quality granular and long-term climate datasets and as well as capability to interpret the same. Actions to this end include integrating resilience thinking within institutional and governance frameworks and defining standards for “high-quality data”. [11]
Investment in non-traditional NWA projects, such as leveraging energy efficiency, demand response, and distributed energy resources (DERs) such as rooftop photovoltaic solar and energy storage, can be also explored as substitutes to traditional costly infrastructure to meet system needs as they may be at lower physical risk.[12] A framework comparing the cost and performance of traditional and non-traditional NWA projects can alleviate reliability and system performance concerns of distribution entities regarding the NWA due to its limited track record as an emergent solution.[13]
Thus, there is immense scope for collaboration and sharing of best practices across geographies to develop a shared understanding of how climate change affects power sector planning and infrastructure and the ways to act on it.
Invest in grid-hardening measures
Actions
- Develop frameworks to identify the risks to physical infrastructure and provide critical grid-hardening policies that can be adopted at the local and national levels to reduce vulnerability
- Catalogue steps taken by utilities in response to climate risks to aid rapid development of grid-hardening plans
- Share updated strategies for improving power sector resilience by upgrading regulations and adequacy mechanisms and processes for the approval of new infrastructure across countries
- Identify sources of low-cost financing to enable a reliable and resilient electricity system
Rationale
Few infrastructure assets are not affected by the risk from climate-associated extreme-weather events. In fact, US utilities may see costs of damage to physical infrastructure increase by 23 percent by 2050.[14] Electricity transmission and distribution lines and substations are especially vulnerable to certain extreme weather conditions. Even renewable generation resources such as solar PV and wind generation infrastructure are susceptible to physical damage and power disruption.[15]
Government regulators should implement strategies to enhance grid resilience, including developing response plans to climate change risks to grid infrastructure. An overarching resilience strategy should include identifying targeted goals to achieve grid-adaptation needs. Utilities must perform need assessments of critical transmission and distribution infrastructure, such as substations. Further, utilities should also develop pathways for long-term grid-hardening based on localised risks. Actions by utilities can be aided by access to open-source information on steps taken by peer organisations internationally.
Figure 2: Energy infrastructure risk

Source: Will Infrastructure Bend or Break Under Climate Stress? (McKinsey Global Institute, 2020) [16]
To mitigate the risk of both financial and physical damage to power infrastructure, power sector planners should identify their vulnerabilities and incorporate relevant actions across all levels of planning and decision making.[19] This includes updating regulations (codes, equipment standards and other guidelines), procurement and contractual processes as well as conducting iterative reviews.[20] Where utilities lack the financial wherewithal to undertake resilience interventions including routine operations and maintenance or large capital investments, the right financing routes must be identified.
The Global Shield Initiative announced at COP27 and coordinated by the G7 can serve as an important template for means to rapidly deploy protection funding.[21] Replicating a similar programme across G20 member states can improve access to low-cost financing needed for grid hardening.
Leverage distributed clean energy technologies and grid digitalisation
Actions
- Engage in collaborative efforts and strategic investments to optimise the deployment of DERs, supported by the implementation of favourable regulatory upgrades
- Share upgrades to policy frameworks that enable DERs and DER aggregators such as virtual power plants (VPPs) to participate in electricity markets
- Share best practices of balancing incentives for DER owners while meeting the needs of grid/power utilities
Rationale
Electricity demand is set to rise across the world, particularly due to increasing per capita electricity demand and electrification of multiple sectors in China and other Asian countries.[22] The buildings and transportation sectors too are taking steps to boost electrification while sourcing electricity from renewable sources where available. Industry, the largest consumer of energy, is gradually increasing the share of electricity used in its processes.
While increasing electrification reduces localised emissions, it also concentrates the risks of widespread disruption on the grid by stressing the existing grid network and generation capacity. Earlier, where a power outage may have affected only access to lighting and powering appliances, it will soon have a bearing on cooking, commuting and industrial productivity, as these sectors transition to an electricity-based energy source to achieve necessary decarbonisation goals (see Figure 3).
Figure 3: Direct and indirect impacts of a climate-induced extreme-weather event
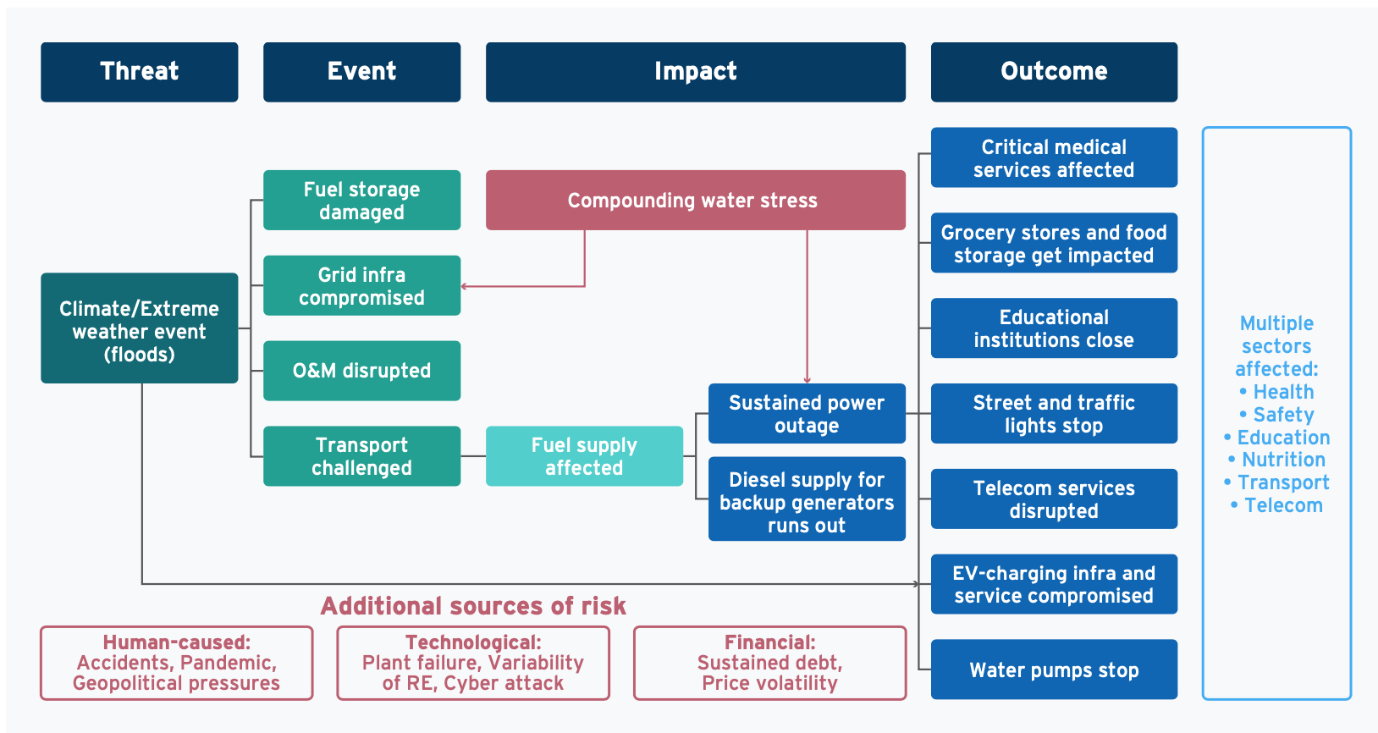
Source: Powering Through: A Climate Resilient Future (RMI and RMI India, 2021)[23]
Decentralisation—diversifying in terms of scale and location while adopting more RE—and digitalisation—moving to ‘smart’ infrastructure for power transmission and distribution—represent lucrative interventions in the power sector due to the value they offer, including, but not limited to, resilience services.
DERs include distributed solar and wind, electric vehicles (EVs), microgrids, energy storage, demand response and energy efficiency.[26] They can integrate RE locally in both grid-connected and off-grid formats, and alleviate the centralisation of risks on the main grid infrastructure. Digitalisation of the electricity sector can support interventions for improved distribution network management. Advanced communication and remote management capabilities through interventions such as smart meters, smart grids and creation of digital twins can help respond to disruptions in real time. For example, microgrids can introduce redundancy into power systems with the process of islanding, wherein microgrids can be disconnected from the main grid during a disruption using intelligent control systems and run independently while generating electricity locally.[27] These functionalities are especially useful for communities vulnerable to extreme weather events.
Departing from the traditional one-way flow of electricity, grid-connected DERs and smart appliances will enable consumers to interact with the grid through two-way power flow and participate in demand flexibility and demand response programmes. These features can help manage load on the grid during both regular operations and disruption. DERs, including rooftop solar, EVs and batteries, can provide emergency backup services. Bundled DERs can offer compounded benefits still. For instance, VPPs can leverage multiple DERs by aggregating latent potential of these assets into a unified portfolio to provide benefits to DER owners and other electricity users on the grid. Through digital networks, VPPs can charge and discharge DERs in response to market signals and grid needs.[28]
However, existing business models and regulations can present challenges in realising the full potential of DERs. Therefore, upgrades to regulatory frameworks, which provide more certainty to DER owners such as on asset ownership and income-generation potential by opening up electricity markets to participation by DERs and aggregated DERs, can help unlock benefits.
Invest in energy storage ecosystems that provide balancing services and flexibility
Actions
- Collaborate on policies that foster enabling environments for rapid deployment of energy storage technologies
- Provide a framework for system assessments to determine storage needs with a key focus on flexibility and explore mechanisms for incentivising resilience through flexibility
- Cooperate to create incentive structures to continue developing innovative storage technologies that cater to various end uses.
Rationale
Energy storage technology is key for successful transitions to low-cost variable RE sources and DERs. Global cumulative installed storage capacity projections are expected to exceed 411 gigawatts by 2030, 15 times of that in 2021.[29] This is understandable given the wide range of services that storage provides, such as shifting load, providing ancillary services for balancing, and restoring operations during blackouts. For utilities, storage can defer or avoid costly infrastructure investments that may be at risk during extreme weather events. For consumers, storage can provide backup power and enable islanding to avoid service disruptions.[30] Many of the services reinforce grid resilience, improve the performance of DERs, and prevent harm or disruption of critical services during times of system failure.
Figure 4: Resiliency cases of battery storage
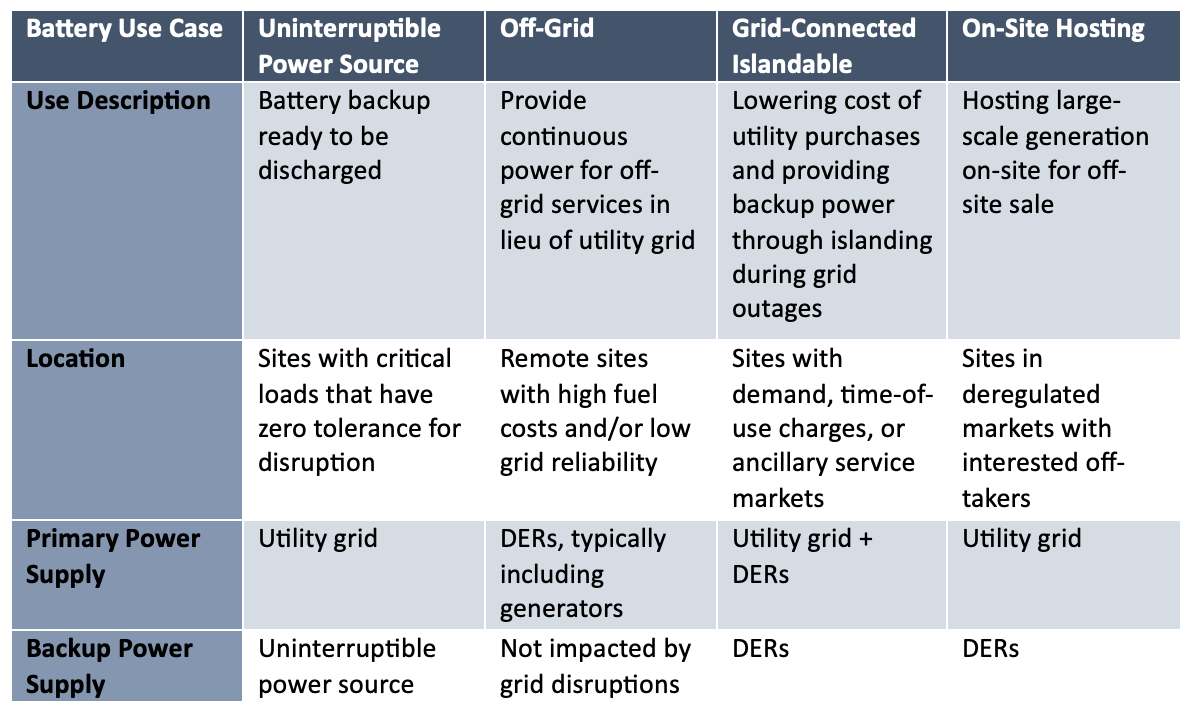
Source: Planning a Resilient Power Sector (Resilient Energy Platform, 2019)[31]
In geographies with liberalised electricity markets, storage deployment can be economically incentivised through market mechanisms that boost resiliency and flexibility services.[33] In addition, G20 member states must consider how to improve finances for more attractive returns and provide clear policies to address and alleviate uncertainty around project timelines.[34]
Incentive structures should be utilised for continued development of innovative storage technologies that cater to various end uses. Technologies that perform in a range of environments with fast response and appropriate discharge durations are critical to improving power system resilience. Targeting development, commercialisation and deployment of new technologies suited for resilience needs ensures that suitable products are in place during disruption.
The production-linked incentive (PLI) scheme for advanced chemistry cell batteries initiated in India provides one framework for incentivising the development of best-fit application technologies. The PLI scheme bolstered the domestic manufacturing of innovative battery technologies by awarding incentives based on key performance criteria. The subsidies were linked to technologies that adhered to these criteria beyond incumbent chemistries being manufactured at scale. The greater an innovative technology performed as per the identified criteria, the higher was the subsidy rewarded.[35] Scoping policies with incentives based on key performance criteria suitable for resiliency ensure that system needs are met.
Attribution: Nuvodita Singh, Benny Bertagnini, and Jagabanta Ningthoujam, “Embedding Resilience in Power Sector Planning,” T20 Policy Brief, June 2023.
[1] Fan Zhang, “In the Dark: How Much Do Power Sector Distortions Cost South Asia,” Economic Times, December, 2018.
[2] Jagabanta Ningthoujam, Nuvodita Singh, and Clay Stranger, “Powering Through: A Climate Resilient Future,” RMI and RMI India, last modified July 2021.
[3] “Electricity Demand per Capita in G20,” Database.earth, accessed May 23, 2023.
[4] International Solar Alliance, accessed March 1, 2023.
[5] “Security of Clean Energy Transitions,” International Energy Agency, accessed May 23, 2023.
[6] “Power Systems in Transition,” International Energy Agency, accessed March 1, 2023.
[7] “Building a Resilient Power Sector,” WBCSD, last modified March 30, 2014.
[8] Elizabeth Weise, “Punishing Heat Wave in India Reaches 115 Degrees, Part of a ‘Hotter and More Dangerous World’,” Phys.Org., May 2, 2022.
[9] Lou Del Bello, “Why India’s Heatwave has Turned Out the Lights,” The Third Pole, May 4, 2022.
[10] WBCSD, “Building a Resilient Power Sector.”
[11] WBCSD, “Resilient Power Sector.”
[12] Cristin Lyons, “Non-Wires Alternatives: Non-Traditional Solutions to Grid Needs,” T&D World, June 6, 2019.
[13] Lyons, “Non-Wires Alternatives.”
[14] Sarah Brody, Matt Rogers, and Giulia Siccardo, “Why, and How, Utilities Should Start to Manage Climate-Change Risk,” McKinsey and Company, last modified April 24, 2019.
[15] Sadie Cox, Eliza Hotchkiss, Dan Bilello, Andrea Watson, Alison Holm, and Jennifer Leisch, “Bridging Climate Change Resilience and Mitigation in the Electricity Sector Through Renewable Energy and Energy Efficiency,” USAID and NREL, last modified November 2017.
[16] Jonathan Woetzel, Dickon Pinner, Hamid Samandari, Hauke Engel, Mekala Krishnan, Brodie Boland, Peter Cooper, and Byron Ruby, “Will Infrastructure Bend or Break Under Climate Stress?” McKinsey Global Institute, last modified August 19, 2020.
[17] Henry Neufeldt, Gerardo Sanchez Martinez, Anne Olhoff, Christian Micheal Skou Knudsen, and Kelly E.J. Dorkenoo, “The Adaptation Gap Report 2018. United Nations Environment Programme (UNEP), Nairobi, Kenya.” DTU, 2018.
[18] “Climate-Resilient Infrastructure.” MIT Climate Portal, last modified September 20, 2021.
[19] WBCSD, “Building a Resilient Power Sector.”
[20] Nathan Lee and Sherry Stout, “Planning a Resilient Power Sector,” Resilient Energy Platform, last modified May 2019.
[21] Kate Abnett and Simon Jessop, “G7 Launches Climate ‘Shield’ Fund, Some Countries Wary,” Reuters, last modified December 2022.
[22] “Renewables Will Be World’s Top Electricity Source Within Three Years, IEA Data Reveals,” World Economic Forum, last modified February 9, 2023.
[23] RMI, “Powering Through: A Climate Resilient Future.”
[24] Erin Douglas, “Texas Largely Relies on Natural Gas for Power. It Wasn’t Ready for the Extreme Cold.” The Texas Tribune, February 16, 2021.
[25] Douglas, “Texas.”
[26] Joseph Stekli, Linquan Bai, Umit Cali, Ugur Halden, and Marthe Fogstad Dynge, “Distributed Energy Resource Participation in Electricity Markets: A Review of Approaches, Modeling, and Enabling Information and Communication Technologies,” Energy Strategy Reviews 43, (September 2022).
[27] “Why Decentralized Systems are the Immediate Path Forward for America’s Energy Goals,” Power, last modified June 27, 2021.
[28] “RMI Launches ‘Virtual Power Plant Partnership’ With Support from General Motors & Google Nest,” RMI, last modified January 10, 2023.
[29] “Global Energy Storage Market to Grow 15-Fold by 2030,” BloombergNEF, last modified October 12, 2022.
[30] Garrett Fitzgerald, James Mandel, Jesse Morris, and Hervé Touati, “The Economics of Battery Energy Storage,” RMI, last modified October 2015.
[31] Resilient Energy Platform “Planning.”
[32] Noel Nevshehir, “These are the Biggest Hurdles on the Path to Clean Energy,” World Economic Forum, last modified February 19, 2021.
[33] “Grid-Scale Storage,” IEA, last modified September 2022.
[34] Aakash Arora, William Acker, Brian Collie, Danny Kennedy, David Roberts, Ian Roddy, James Greenberger, et al., “How Lithium Batteries Can Power the US Economy,” BCG, last modified February 24, 2023.
[35] Randheer Singh, Akshima Ghate, Jagabanta Ningthoujam, Arjun Gupta, Shashwat Sharma, and Benny Bertagnini, “Need for Advanced Chemistry Cell Energy Storage in India,” RMI, NITI Aayog, last modified April 2022.